Coal provides the world’s principal source of primary energy for generating electrical base load power. However, the inherently polluting nature of pulverised coal combustion drives major research efforts to gain a deeper understanding of its underlying chemical and physical processes, such that pollution and climate change can be mitigated. This projects aims to develop a multi-dimensional flamelet model for pulverised coal combustion, which can be used as an efficient sub-grid model for LES. The project is split into three parts, which are distributed among three universities, namely the TU Darmstadt, the University of Stuttgart and the University of Duisburg-Essen. The subprojects consider the key competence of the project partners; a) the development of the flamelet model (Darmstadt); b) the a priori simulation and validation by direct numerical simulation (Stuttgart) and c) the a posteriori application in LES (Duisburg-Essen). The flamelet model considers two fuel streams from the particles, namely volatile gases from pyrolysis and char-off gases from char conversion. The flamelet method is developed and validated based on DNS of reacting coal particles in both laminar and turbulent flows for single particle and group combustion. The results from flamelet modelling and DNS guide the development of a new comprehensive LES model for the simulation of thermal coal conversion.
While phase 1 of the project focused on improving our fundamental understanding of the basic coal combustion physics, current phase 2 has set a focus on pollutant formation, namely NOx and SOx.
Movie 1
Movie 1: Fully-resolved simulations of multiple coal particle devolatilisation and volatile flame interaction in laminar flow. The flow direction is from left to right and arrays of three layers of particles arranged axially with periodic boundary conditions in the two cross-stream directions are simulated (infinite particle layers), with a relative inter-particle spacing of 5 particle diameters (top), 10 diameters (middle) and 25 diameters (bottom). The top half of each animation shows the OH radical mass fraction (orange/yellow = high) as a marker for the reaction zone. The bottom half shows the O2 mass fraction (yellow = high) as an indicator for the availability of oxidiser to convert the volatiles. The green, black and white lines represent the iso-surfaces of twice, one time and half the stoichiometric mixture fraction, i.e. the black lines mark stoichiometric volatile/air mixtures. Transient simulations are performed, where the particles are heated up first, followed by devolatilisation, volatile flame ignition and burning, and finally extinction, whereas char conversion is not included here. The interaction behaviour of the volatile flames is a strong function of the particle Reynolds number Rep and the relative inter-particle spacing, where Rep = 2 for all three cases.
For an inter-particle spacing of 5 diameters (top) the flames strongly interact and at the time of the peak devolatilisation rate (here: 4.1ms) total group combustion is observed, where a single flame sits at the front of the particle array and no oxygen is left at the interior of the particle array. When increasing the relative spacing to 10 diameters (middle) partial group combustion is observed at the time of maximum devolatilisation rate (here: 4.4ms), such that the particles in the two front layers of the array are enveloped by a joint flame, whereas the final particle layer is starved of oxygen. When further increasing the spacing to 25 particle diameters (bottom) isolated particle combustion is observed at the time of maximum devolatilisation rate (here: 4.5ms), where individual volatiles flames are formed around the particles in each layer. More details can be found in Tufano et al., Fuel 229 (2018) 262-269.
Movie 2
Movie 2: Similar simulation as for Movie 1, but with a large particle Reynolds number (Rep = 8) and for a relative particle spacing of 10 diameters. The movie shows the OH mass fraction only. Due to the higher Rep (at equivalent spacing) compared with Movie 1 (middle), the front layer of particles can only sustain weak volatile flames in its wake, whereas the two downstream layers interact strongly in axial direction and weakly in the cross-stream directions. An array of 3x3x3 particles is used for this laminar case to be able to make direct comparisons to turbulent flow conditions (see Movie 3). More details can be found in Tufano et al., Fuel 234 (2018) 723-731.
Movie 3
Movie 3: Similar simulation as for Movie 2 (same Rep and relative spacing), but now with turbulent fluctuations superimposed on the flow coming in from the left. It can be observed that turbulence leads to strong interactions between the volatile flames of neighbouring particles, which act to weaken (flames in the two downstream layers) or enhance (flames in the front layer) the volatile flames at different times during the simulation. More details can be found in Tufano et al., Fuel 234 (2018) 723-731.
Movie 4
Movie 4: Carrier-phase DNS (CP-DNS) of detailed NOx formation in early-stage pulverized coal combustion with fuel-bound nitrogen. (Left:) OH and (right:) NO mass fraction shown in a 2D slice extracted from the 3D computational domain. The fuel particles are shown in grey. The white lines at late times are iso-lines of stoichiometric volatile mixture fraction.
A temporally evolving mixing layer is created by initially dividing the domain into two counter-flowing streams, where each stream occupies half of the cross-stream width (y-direction). The mean velocities of the upper and lower stream are 2.4 m/s and -17.6 m/s in streamwise (x) direction, respectively. The upper stream consists of preheated air at T=500 K, while the lower stream consists of a mixture of volatiles and air at chemical equilibrium with T=1600 K and a volatile mixture fraction of Z=0.0398. A cloud of 300,000 Lagrangian particles, with an initial diameter of 25 micron is distributed randomly in the upper stream.
The CP-DNS setup aims at simulating the processes that occur at the early stages of PCC in a typical coal furnace, namely heating of the cloud of particles due to turbulent mixing, devolatilization, ignition, char conversion and volatile combustion, all of which can be observed in the animation.
This particular simulation sets its focus on the detailed prediction of NOx. NOx is a hazardous pollutant the release of which needs to be avoided, while its formation processes from coal are partially unknown. Here, we employ a comprehensive NOx formation model that includes all known chemical routes of NOx formation in the gas phase, along with a newly developed detailed model for NOx formation from fuel-bound nitrogen based on pyridine. More details can be found in Shamooni et al., Fuel 291 (2021) 119998.
Movie 5
Movie 5: Flamelet/progress variable modelling for large eddy simulation (LES) of turbulent pulverised coal combustion in the swirling gas-assisted solid fuel combustion chamber of TU Darmstadt. The six-dimensional flamelet table considers the mixing of the pilot, volatile and char off-gas fuel streams with air, as well as chemical reaction progress, interphase heat transfer and sub-grid scale variations. Left: Temperature contour along a horizontal plane crossing the centreline of the burner. Right: Pulverised coal particles coloured by their temperature. The animation begins when the interior of the furnace has been heated up by single-phase gas combustion of the pilot fuel such that coal particles can be injected for their subsequent heat-up, devolatilisation, volatile combustion and char conversion, i.e. the establishment of the turbulent reacting multiphase flow.
Related publications
- X. Wen, A. Shamooni, H. Nicolai, O. T. Stein, A. Kronenburg, A. M. Kempf, and C. Hasse, “Flame structure analysis and flamelet modeling of turbulent pulverized solid fuel combustion with flue gas recirculation,” Proc. Combust. Inst., (2023).
- T. D. Luu, A. Shamooni, O. T. Stein, A. Kronenburg, S. Popp, H. Nicolai, H. Schneider, X. Wen, and C. Hasse, “Flame characterisation of gas-assisted pulverised coal combustion using FPV-LES,” Proc. Combust. Inst., (2023).
- A. Shamooni, O. T. Stein, A. Kronenburg, A. M. Kempf, P. Debiagi, T. Li, A. Dreizler, B. Böhm, and C. Hasse, “Fully-resolved simulations of volatile combustion and NOx formation from single coal particles in recycled flue gas environments,” Proc. Combust. Inst., (2023).
- J. Xing, K. Luo, Y. Chen, O. T. Stein, A. Kronenburg, K. H. Luo, C. Hasse, and J. Fan, “Large eddy simulation of Cambridge bluff-body coal (CCB2) flames with a flamelet progress variable model,” Proc. Combust. Inst., vol. 38, pp. 5347–5354, (2021).
- T. D. Luu, A. Shamooni, O. T. Stein, A. Kronenburg, S. Popp, H. Nicolai, H. Schneider, X. Wen, and C. Hasse, “Analysis of heat transfer effects in flamelet/progress variable LES of gas-assisted pulverised coal flames,” in 30. Deutscher Flammentag 2021, 28-29 Sept, Hannover-Garbsen, Germany, (2021).
- D. Meller, T. Lipkowicz, M. Rieth, O. T. Stein, A. Kronenburg, C. Hasse, and A. M. Kempf, “Numerical Analysis of a Turbulent Pulverized Coal Flame Using a Flamelet/Progress Variable Approach and Modeling Experimental Artifacts,” Energy & Fuels, vol. 35, pp. 7133–7143, (2021).
- X. Wen, H. Nicolai, O. T. Stein, J. Janicka, A. Kronenburg, and C. Hasse, “Effects of air and oxy-fuel atmospheres on flamelet modeling of pollutant formation in laminar counterflow solid fuel flames,” Fuel, vol. 285, p. 119079, (2021).
- B. Wang, A. Shamooni, O. T. Stein, A. Kronenburg, A. M. Kempf, P. Debiagi, and C. Hasse, “Investigation of Turbulent Pulverized Solid Fuel Combustion with Detailed Homogeneous and Heterogeneous Kinetics,” Energy & Fuels, vol. 35, pp. 7077–7091, (2021).
- X. Wen, A. Shamooni, O. T. Stein, A. Kronenburg, L. Cai, H. Pitsch, A. M. Kempf, and C. Hasse, “Detailed analysis of early-stage NOx formation in turbulent pulverized coal combustion with fuel-bound nitrogen,” Proc. Combust. Inst., vol. 38, pp. 4111–4119, (2021).
- A. Shamooni, P. Debiagi, B. Wang, T. D. Luu, O. T. Stein, A. Kronenburg, G. Bagheri, A. Stagni, A. Frassoldati, T. Faravelli, A. M. Kempf, X. Wen, and C. Hasse, “Carrier-phase DNS of detailed NOx formation in early-stage pulverized coal combustion with fuel-bound nitrogen,” Fuel, vol. 291, p. 119998, (2021).
- X. Wen, M. Rieth, A. Scholtissek, O. T. Stein, H. Wang, K. Luo, A. Kronenburg, J. Fan, and C. Hasse, “A comprehensive study of flamelet tabulation methods for pulverized coal combustion in a turbulent mixing layer—Part II: Strong heat losses and multi-mode combustion,” Combust. Flame, vol. 216, pp. 453–467, (2020).
- X. Wen, M. Rieth, A. Scholtissek, O. T. Stein, H. Wang, K. Luo, A. M. Kempf, A. Kronenburg, J. Fan, and C. Hasse, “A comprehensive study of flamelet tabulation methods for pulverized coal combustion in a turbulent mixing layer — Part I: A priori and budget analyses,” Combust. Flame, vol. 216, pp. 439–452, (2020).
- G. L. Tufano, O. T. Stein, A. Kronenburg, G. Gentile, A. Stagni, A. Frassoldati, T. Faravelli, A. M. Kempf, M. Vascellari, and C. Hasse, “Fully-resolved simulations of coal particle combustion using a detailed multi-step approach for heterogeneous kinetics,” Fuel, vol. 240, pp. 75–83, (2019).
- X. Wen, O. T. Stein, P. Debiagi, A. Scholtissek, A. Kronenburg, A. M. Kempf, and C. Hasse, “Detailed simulations for flamelet modelling SOx formation from coal,” in Proc. Appl. Math. Mech., 24th May 2019, Vienna, Austria, (2019).
- X. Wen, O. T. Stein, G. L. Tufano, A. Kronenburg, A. Scholtissek, and C. Hasse, “Multi-dimensional and transient effects on flamelet modeling for turbulent pulverized coal combustion,” Fuel, vol. 255, p. 115772, (2019).
- M. Rieth, A. M. Kempf, O. T. Stein, A. Kronenburg, C. Hasse, and M. Vascellari, “Evaluation of a flamelet/progress variable approach for pulverized coal combustion in a turbulent mixing layer,” Proc. Combust. Inst., vol. 37, pp. 2927–2934, (2019).
- X. Wen, P. Debiagi, O. T. Stein, A. Kronenburg, A. M. Kempf, and C. Hasse, “Flamelet tabulation methods for solid fuel combustion with fuel-bound nitrogen,” Combust. Flame, vol. 209, pp. 155–166, (2019).
- G. L. Tufano, O. T. Stein, B. Wang, A. Kronenburg, M. Rieth, and A. M. Kempf, “Coal particle volatile combustion and flame interaction. Part I: Characterization of transient and group effects,” Fuel, vol. 229, pp. 262–269, (2018).
- G. L. Tufano, O. T. Stein, B. Wang, A. Kronenburg, M. Rieth, and A. M. Kempf, “Coal particle volatile combustion and flame interaction. Part II: Effects of particle Reynolds number and turbulence,” Fuel, vol. 234, pp. 723–731, (2018).
- Y. Chen, O. T. Stein, A. Kronenburg, M. Vascellari, C. Hasse, and K. H. Luo, “Flamelet progress variable modelling of pulverized coal devolatilisation and burning in opposed jets,” in Conf. on Modelling Fluid Flow (CMFF), Budapest, Hungary., (2018).
- M. Rieth, A. M. Kempf, A. Kronenburg, and O. T. Stein, “Carrier-phase DNS of pulverized coal particle ignition and volatile burning in a turbulent mixing layer,” Fuel, vol. 212, pp. 364–374, (2018).
- M. Vascellari, G. L. Tufano, O. T. Stein, A. Kronenburg, A. M. Kempf, A. Scholtissek, and C. Hasse, “A flamelet/progress variable approach for modeling coal particle ignition,” Fuel, vol. 201, pp. 29–38, (2017).
- M. Rieth, A. G. Clements, M. Rabaçal, F. Proch, O. T. Stein, and A. M. Kempf, “Flamelet LES modeling of coal combustion with detailed devolatilization by directly coupled CPD,” Proc. Combust. Inst., vol. 36, pp. 2181–2189, (2017).
- G. L. Tufano, O. T. Stein, R. Knappstein, J. Janicka, and A. Kronenburg, “Resolved flow simulation of pulverized coal particle combustion past a premixed flame front,” in Proc. 8th Europ. Combust. Meeting, Dubrovnik, Croatia, (2017).
- G. L. Tufano, O. T. Stein, A. Kronenburg, A. Frassoldati, T. Faravelli, L. Deng, A. M. Kempf, M. Vascellari, and C. Hasse, “Resolved flow simulation of pulverized coal particle devolatilization and ignition in air- and O2/CO2-atmospheres,” Fuel, vol. 186, pp. 285–292, (2016).
- G. L. Tufano, C. Hasse, M. Vascellari, A. M. Kempf, I. Wlokas, L. Deng, A. Kronenburg, and O. Stein, “Direct numerical simulation of coal particle devolatilization and volatile combustion,” in 7th European Combustion Meeting, 7th European Combustion Meeting, (2015).
Contact

Oliver T. Stein
Dr.former deputy director
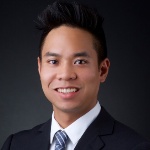
Tien Duc Luu
Ali Shamooni
Dr.-Ing.Scientific staff